On January 20, Chinese scientists reported that they were able to maintain a plasma at a temperature of 100 million degrees C for about 1,066 seconds in a nuclear fusion reactor called the Experimental Advanced Superconducting Tokamak (EAST).
In 1938, physicists Otto Hahn and Fritz Strassmann found that energy is produced when the nucleus of an atom breaks apart, a process that Lise Meitner and Otto Frisch explained a year later as a process called ‘fission’. Only four years later, physicists used this principle to build and operate the world’s first reactor with a sustainable nuclear fission reaction.
By this time physicists also knew that energy is also produced when two atomic nuclei fuse together, a process called fusion. Nuclear fission produces harmful radioactive waste whereas nuclear fusion doesn’t. This is why developing a nuclear fusion reactor has become an important technological goal for a world keenly interested in new sources of clean energy.
The tritium problem
The problem is the amount of energy required to start and sustain a fusion reaction. A nuclear fission reaction can be kicked off by shooting neutrons of suitable energy at the atoms of unstable nuclei like uranium. For fusion to occur, however, the nuclei need to be exposed to a temperature of at least 100 million degrees C.
The lightest nucleus in nature is of hydrogen, consisting of a single proton. An isotope of hydrogen called deuterium has one proton and one neutron in its nucleus. The nucleus of another isotope called tritium has one proton and two neutrons. Deuterium-deuterium fusion requires a higher temperature to begin than deuterium-tritium fusion. This is because the extra neutron in the tritium nucleus helps overcome the repulsion of like-charges between the protons.
The fusion of a deuterium and a tritium nucleus creates a non-radioactive helium-4 nucleus, a neutron, and 17.6 MeV of energy, which is significant. The neutron can be directed to a blanket of materials surrounding the reactor that capture it and release more heat.
While deuterium is abundant in seawater, there are no natural deposits of tritium and it is very hard to produce. At present it is mostly created as a by-product in heavy-water fission reactors in Canada, India, and South Korea.
The temperature problem
Yet another challenge for nuclear fusion is the temperature. For two nuclei to fuse, two things need to happen: the like-charges in the nuclei (due to the protons) need to be overcome, then the particles need to come within around 1 femtometre (fm) of each other so they can bond with each other using the strong nuclear force.
This force is the strongest fundamental force in nature and is responsible for keeping protons and neutrons together in the nuclei of atoms. But on the flip side, it only acts across very short distances: 1 fm is roughly one-fourth the width of a carbon nucleus. This is why the nuclei need to be heated to such high temperatures: to give them enough energy to overcome their repulsion and get so close to each other.
There are different reactor designs to achieve nuclear fusion by meeting these conditions. One set of designs involves the use of a tokamak — a donut-shaped vessel where the nuclei are confined, like in a cage, and made to fuse.
A magnetic cage
Inside the vessel, a deuterium gas is exposed to about 20 million degrees C, when matter exists in the plasma state. The charged particles are stripped from their respective atoms and float around freely. Next, the particles are exposed to a very strong magnetic field that acts like an invisible net, trapping the particles along the field lines. This method is called magnetic confinement.
Engineers prefer to use electromagnets — special materials that generate a magnetic field when a current is passed through wires coiled around them — to create these fields because the field strength only depends on the amount of current in the wires. These wires are also superconducting: they can carry electric current with zero resistance if they are cooled to a very low temperature, which is achieved by blanketing them with liquid nitrogen or helium.

Inside EAST, both toroidal and poloidal magnetic fields are generated by superconducting electromagnets. It is currently the world’s only tokamak with this feature. Toroidal magnetic fields flow around the donut-shaped reactor while poloidal fields flow through its centre. Together, they keep the particles within from drifting into the vessel’s walls and collapsing the plasma. Instead they move in a spiral pattern through the vessel, with opportunities to collide with each other and fuse.
The poloidal field also induces an electric current in the plasma. When parts of the plasma resist the flow of this current, heat is produced, adding to the energy required to achieve fusion.
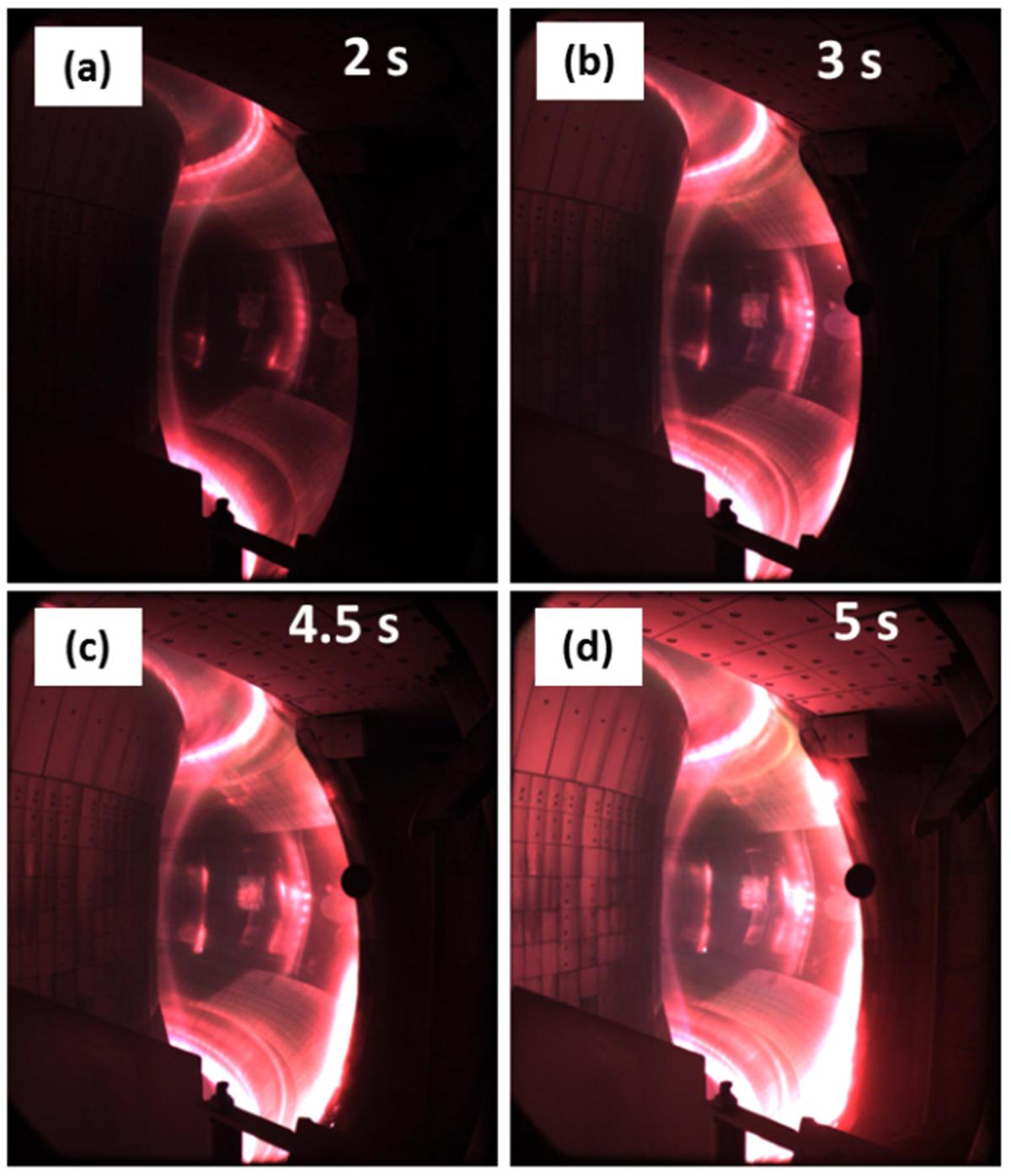
A fast visible wavelength camera’s images of plasma inside the EAST Tokamak at various times.
| Photo Credit:
DOI:10.1088/1741-4326/aa626c
Series of records
Through the years, EAST has been setting a series of records and validating the technologies used to achieve them. It was the first tokamak to sustain a plasma in high-confinement mode at around 50 million degrees C for more than 60 seconds in 2016 and for more than 100 seconds in 2017. In 2023, EAST achieved the world’s first steady-state high-confinement plasma for 403 seconds — a world record that it broke on January 20, 2025, by sustaining a plasma for 1,066 seconds. For this achievement, operators provided twice the thermal power to EAST as they did for the 2023 feat, allowing the plasma to remain stable for longer.
At present, EAST isn’t producing electricity. In fact, it is yet to reach a milestone called ignition: meaning it doesn’t produce enough heat for more fusion reactions to occur, a.k.a. become self-sustaining. To produce usable electricity, a tokamak needs to maintain millions of degrees C for at least a few hours.
EAST is a testbed reactor for ITER, an international megaproject in which six countries around the world, including India, and the European Union are working together to build a tokamak that will sustain nuclear fusion that releases more energy than that required to sustain the plasma.
A need for alternatives
Crucially, EAST’s successes are important for ITER’s future because the latter has come under criticism for its delayed timelines and cost overruns. With a bill already upwards of EUR 18 billion, ITER has been called the most expensive science experiment in history — at a time in which the high cost of doing cutting-edge science has put off many governments from pursuing it.
Some research groups have also been trying to achieve nuclear fusion using methods that require fewer (but still considerable) resources. For example, one alternative to achieving magnetic confinement is a device called a stellarator. Whereas a tokamak has a simple donut shape, a stellarator has a more twisting design that is harder to build and operate. But its advantage is that it does away with the need for a poloidal magnetic field to achieve a twisting magnetic field inside the vessel. Instead it achieves the desired field configuration using a more complicated architecture of external magnets.
Other designs do away with magnetic confinement altogether. In one technique, for example, a pellet of deuterium and tritium is hit with laser beams of extreme power. Whereas a deuterium nucleus has one proton and one neutron, a tritium nucleus has one proton and two neutrons. When the beams strike the pellet, the energy causes the nuclei to compress and fuse, releasing more energy. The heat from the reactions could then be diverted to a pool of water, generating steam that moves a turbine and produces electricity.

In the light of lasers
In 2008, scientists at the Lawrence Livermore National Laboratory in the US began a project called ‘Laser Inertial Fusion Energy’ (LIFE) to test this idea. While they were able to develop lasers with the requisite power, the fusion output was found to be much lower than they had predicted. The project was cancelled in 2013 after it became clear it couldn’t achieve ignition.
But another project at the same institute, called the National Ignition Facility (NIF), achieved the milestone in 2022. At the NIF, a system of 192 high-power lasers delivers 2.05 megajoules (MJ) of energy towards a small cylindrical capsule at the centre of the room. This capsule, called a hohlraum, is made of uranium-238 and plated with gold. It’s about 2 mm wide. It contains a thin shell made of a polymer inside which deuterium and tritium atoms are placed in a frozen or gaseous state.
When electromagnetic radiation from the lasers enters the hohlraum, it strikes the inner wall and produces X-rays. Over a short span of time, the nuclei are bombarded by X-rays from all directions inside the hohlraum. Eventually they compress the fuel sample in a symmetric way and heat it up rapidly to around 100 million degrees C. In 2022, the NIF said it had used this technique to produce 3.15 MJ of energy, crossing the breakeven point.
On the other hand, ITER was launched in 2007 and is expected to produce its first plasma only in 2033, and over time also devour the world’s meagre tritium reserves. The desperate need for sources of clean energy means achieving nuclear fusion may just be a matter of time, especially if governments continue to trust the scientists working on the required technologies. But which technology gets it over the line — magnetic, inertial or something else — remains to be seen. Some private sector enterprises are also beginning to enter the mix.
While the NIF has demonstrated a proof-of-concept ignition, EAST is keeping tokamaks in the hunt with its large scale and steady progress.
Shamim Haque Mondal is a researcher in the Physics Division, State Forensic Science Laboratory, Kolkata.
Published – February 18, 2025 05:30 am IST